Pulsar Wind Nebulae
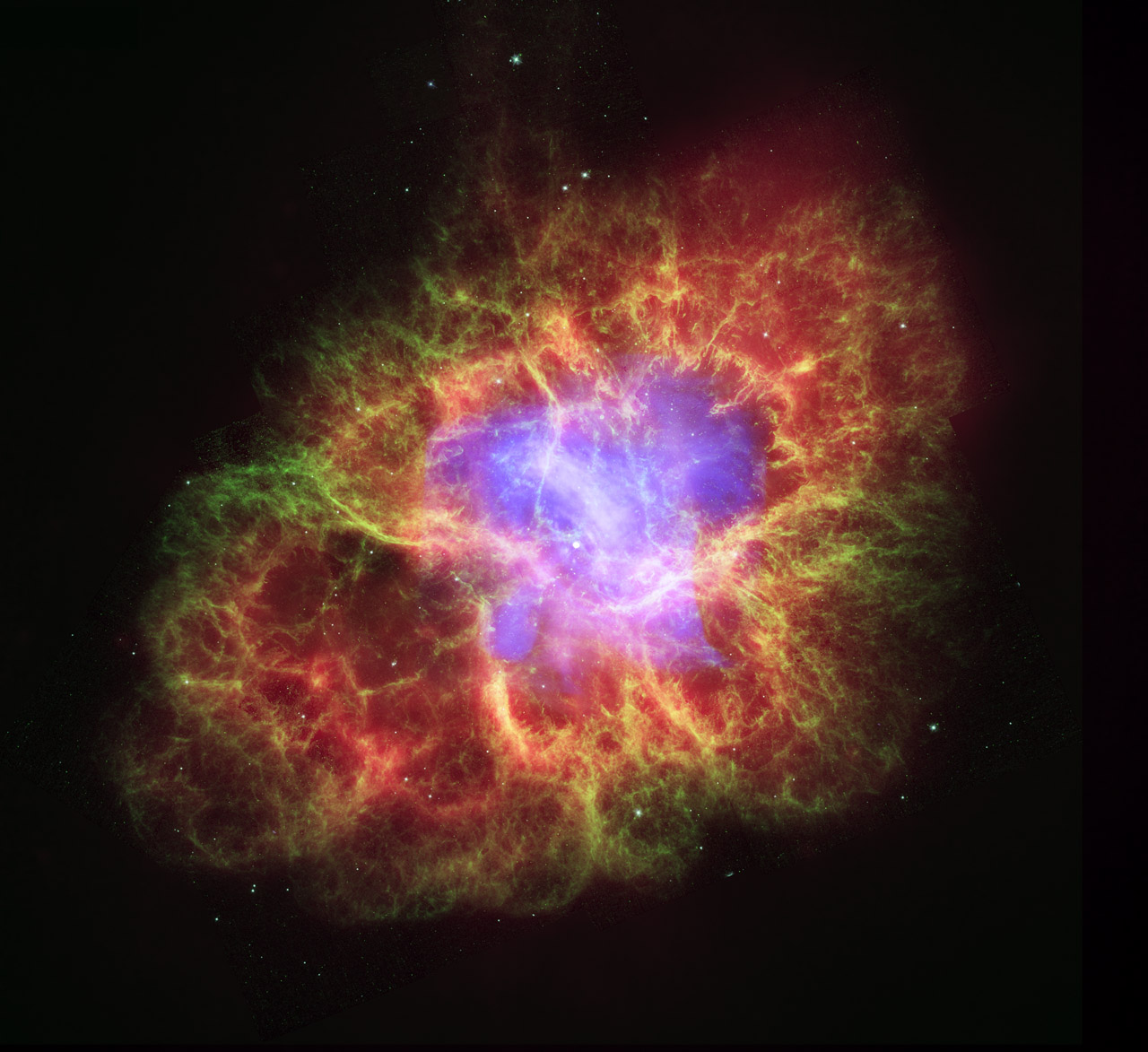
Multi-frequency image of the Crab Pulsar Wind Nebula by the Hubble, Chandra and Spitzer space telescopes. Credit: NASA, ESA, CXC, JPL-Caltech, J. Hester and A. Loll (Arizona State Univ.), R. Gehrz (Univ. Minn.), and STScI
Once a massive star has had its final showdown in a super-nova explosion, it leaves behind an extremely dense remnant: matter is packed so tight as if it was forming a single atomic nucleus. Given enough mass of the compact remnant (around two solar masses), it can even collapse further to form a black hole.
Neutron stars (the remnants which don't become black holes) are particularly interesting astrophysical objects: they are stabilized against ultimate gravitational collapse by the strong nuclear force. Thus studying their structure, we can learn about the fundamental physics of matter at extreme densities.
Many rapidly spinning neutron stars (performing up to a thousand turns every second) are endowed with strong magnetic field and emit pulses of electromagnetic radiation with every one of their turns. These are called Pulsars.
Not only do Pulsars emit regular bursts of electromagnetic radiation, their rotating magnetosphere also drives a relativistic outflow of electrons and positrons, called a Pulsar Wind. Similar to the solar wind, the Pulsar Wind is accelerated by the electromagnetic field, but since its density is so low, it reaches highly relativistic speeds (some models predict Lorentz factors of 105, much faster than any other outflow in the Universe). Once this outflow has expanded for around nine orders of magnitude, its dynamic pressure drops below the pressure of the supernova ejecta and a shock forms -- the wind termination shock. At this shock, the particles of the wind are accelerated and non-thermal radiation illuminates the stellar ejecta from the inside out: a Pulsar Wind Nebula is born.
The most celebrated Pulsar Wind Nebula is the Crab Nebula at a distance of 2000 pc. High resolution optical and X-ray observations have revealed the intricate dynamics of the termination shock: the emergence of a 'jet and torus' morphology, emission of wisp-like perturbations in the flow and the appearance of a 'knot' of emission attached to the shock are only a few of the variable features.
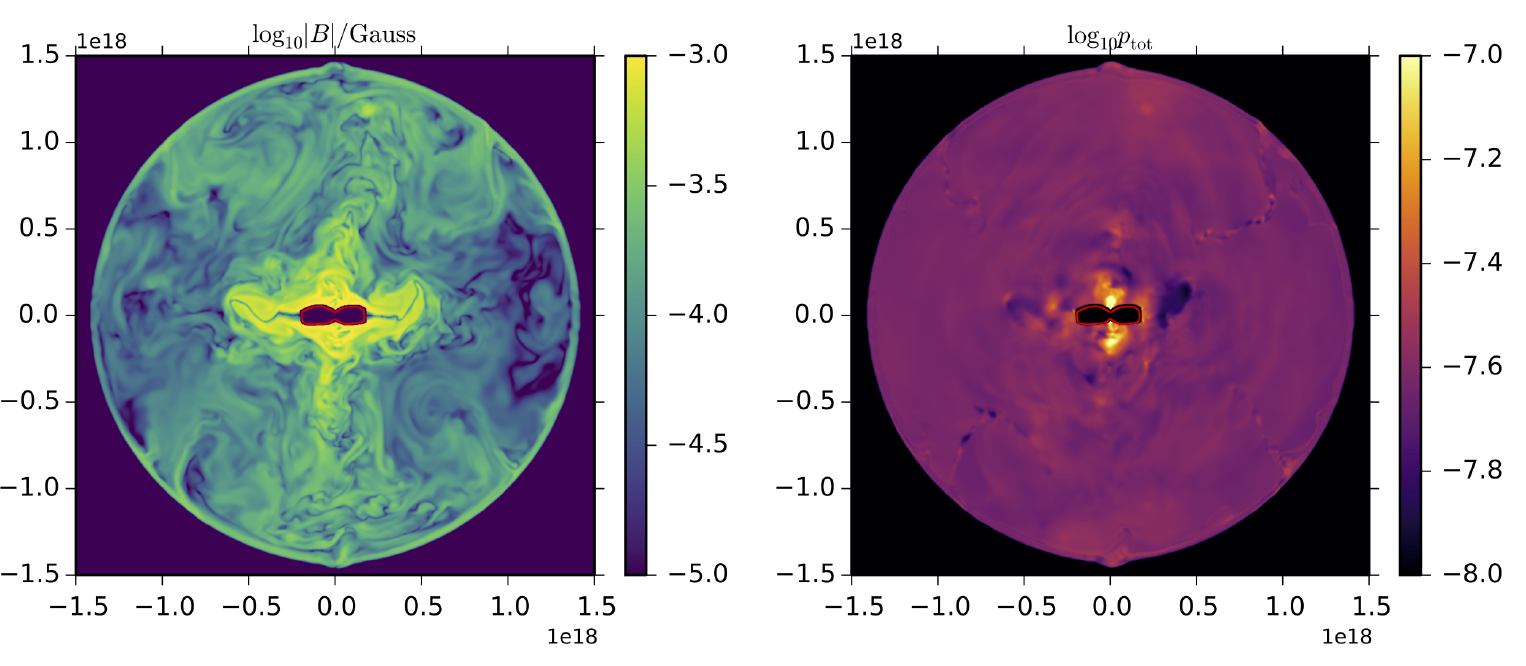
3D simulation of the flow in the PWN showing magnetic field strength (left) and the total pressure in the nebula (right), formation of current sheets, turbulence and dissipation in the nebula dramatically alter the dynamics of the flow. See Three-dimensional magnetohydrodynamic simulations of the Crab nebula for further info.
Pulsar Wind Nebulae are ideal laboratories for relativistic plasma dynamics and their modeling is connected to many questions of astro- and fundamental- physics, for example:
- How does non-thermal particle acceleration take place? How does the particle spectrum evolve in the nebula?
- How is magnetic energy transported to relativistic particles? How does turbulent magnetic dissipation operate?
- What is the origin of gamma-ray flares within the Crab nebula?
- What is the origin of radio-emitting electrons? And how many particles can be supplied by the neutron star magnetosphere?
- How many leptons escape into the ISM and find their way to Earth? Can PWN explain the observed positron excess compared to cosmological predictions?
Porth, Oliver, Buehler, Rolf, Olmi, Barbara, Komissarov, Serguei, Lamberts, Astrid, Amato, Elena, Yuan, Yajie, Rudy, Alexander; Modelling Jets, Tori and Flares in Pulsar Wind Nebulae, Space Science Reviews, Volume 207, Issue 1-4, pp. 137-174
and in a number of works I have carried out over the years, see also Publications.
17th Jun 2019; last edit 6th Jul 2019 by Oliver
tags: website, pwn